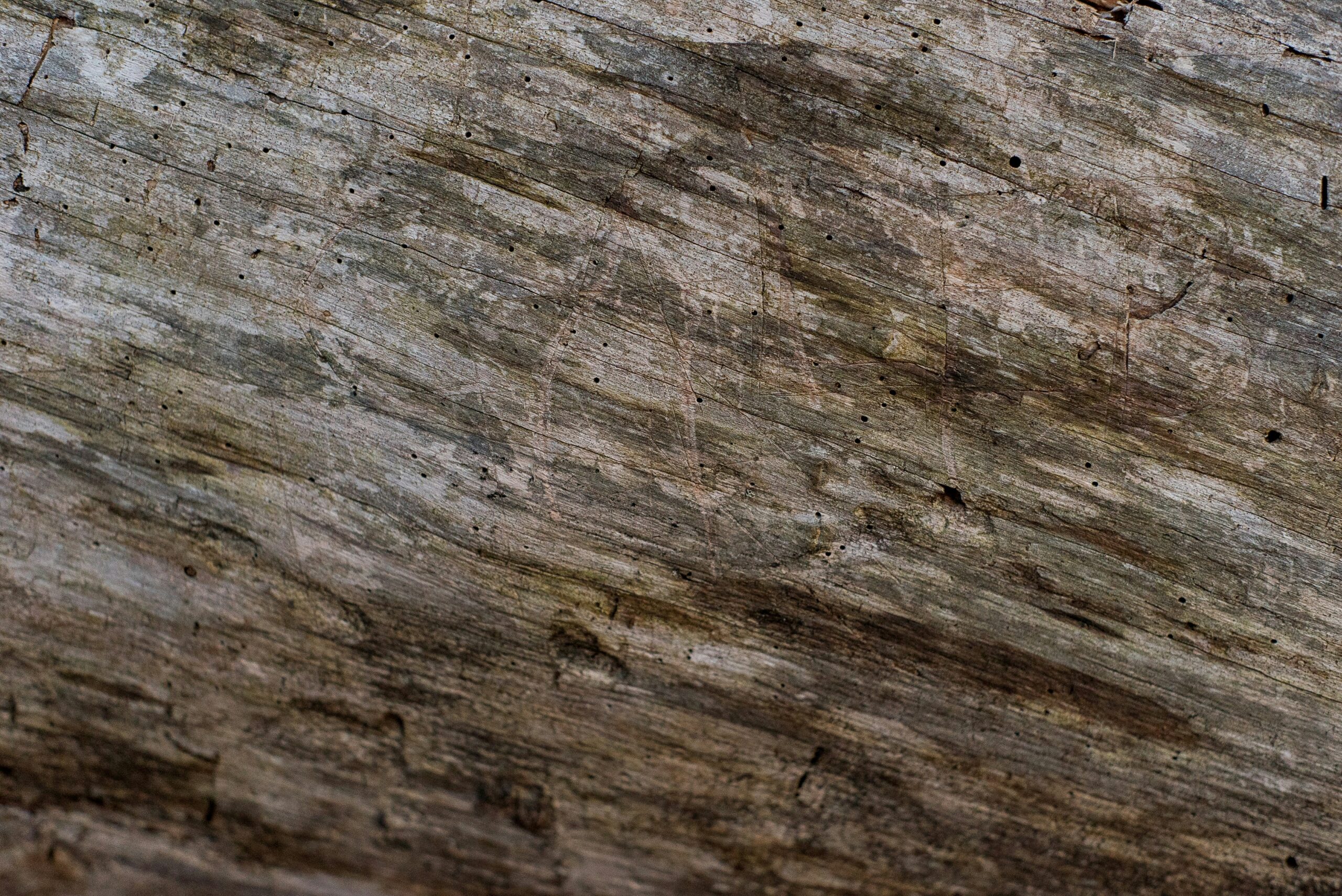
Introduction to the Age of the Earth
Understanding the age of the Earth is a fundamental aspect of geology and the biological sciences. The Earth, as we know it, is approximately 4.5 billion years old, a figure established through various scientific methods that reveal the planet’s formation and the timeline of its development. This remarkable age highlights the intricate geologic processes that have shaped the Earth and the evolution of life over extensive periods.
The significance of knowing the Earth’s age cannot be overstated; it provides context for the history of our planet and its life forms. Various geological and biological processes, such as plate tectonics, erosion, and evolution, are better understood through this temporal lens. Knowledge of the Earth’s age aids scientists in reconstructing the history of the planet, allowing them to study past climates, ancient ecosystems, and significant biological events, such as mass extinctions and the emergence of new species.
To determine the Earth’s age, scientists employ several methods. Radiometric dating techniques, particularly uranium-lead dating, are among the most reliable. This approach measures the decay of radioactive isotopes within rocks and minerals, providing insights into when they formed. Other techniques include stratigraphy, which examines the layered arrangement of rocks, and paleomagnetism, which studies the magnetic properties of rocks to infer the temporal order of geological events.
In this blog post, we will delve deeper into the methods used to ascertain the Earth’s age, the significance of this knowledge in understanding geological and biological processes, and the events that defined its extensive history. By exploring these themes, we will enhance our appreciation for the complexity and intricacy of our planet’s past and present.
Historical Perspectives on Earth’s Age
The quest to determine the Earth’s age has captivated humanity for millennia, beginning with ancient civilizations that sought explanations for the world around them. Early cultures often attributed the origin of Earth to divine creation, as seen in various mythologies, where the age of the planet was linked to spiritual beliefs rather than empirical evidence. For instance, ancient Greeks like Hesiod and Plato proposed creation stories but were limited in their understanding of geological processes.
By the Renaissance, a shift occurred as scientists began to approach the question more systematically. Figures such as Nicolas Copernicus and Galileo Galilei laid the groundwork for a scientific method, encouraging the observation of natural phenomena. However, it was not until the 17th century that individuals like James Ussher, an Anglican bishop, attempted to calculate the Earth’s age through biblical genealogies, famously determining it to be about 6,000 years old. This perspective dominated until the emergence of modern geology.
The 18th and 19th centuries ushered in a new era of research, as geologists like James Hutton and Charles Lyell introduced concepts like uniformitarianism, positing that Earth’s features were shaped over vast timescales by natural processes. This paradigm shift fundamentally changed perceptions of time and allowed for estimates of Earth’s age to extend into millions of years. The advent of radiometric dating techniques in the 20th century, notably through the work of scientists such as Arthur Holmes, provided a more precise method for calculating the Earth’s age. By measuring the decay of radioactive isotopes, researchers concluded that the Earth is approximately 4.5 billion years old.
As our understanding has evolved, it has become clear that the Earth’s age is not merely a number but a reflection of intricate processes spanning billions of years. The historical perspectives on Earth’s age showcase humanity’s journey from mythological interpretations to empirical, scientific inquiry—an ongoing evolution that continues to shape our comprehension of the planet we inhabit.
Scientific Methods for Dating the Earth
Determining the age of the Earth is a complex task that scientists undertake using several scientific methods. Among these, radiometric dating stands out as a key technique. This method relies on the principle of radioactive decay, which occurs when unstable isotopes transform into stable ones over time. By measuring the ratio of parent isotopes to daughter products within a sample, scientists can calculate the age of rocks and fossils with remarkable accuracy. Common isotopes used in radiometric dating include carbon-14 for organic materials and uranium-238 for older geological samples, allowing researchers to construct a timeline for Earth’s history.
Another critical approach is stratigraphy, which involves studying rock layers (strata) and their sequencing. By understanding the relative positions of these layers, geologists can infer the chronological order of events that shaped the Earth. This method is particularly useful for decoding the sedimentary history of specific regions, as the arrangement of sedimentary rocks can provide insights into ancient environments and life forms present at various periods. The principle of superposition, which states that in an undeformed sequence of sedimentary rocks, the oldest layers are at the bottom, further aids in establishing a timeline.
In addition to radiometric dating and stratigraphy, paleomagnetism offers another avenue for determining the age of geological formations. This method examines the magnetic properties of rocks that have recorded the Earth’s magnetic field at the time of their formation. By comparing these records with known shifts in Earth’s magnetic poles, scientists can date the rocks and gain insights into tectonic activity over millions of years.
Through these multifaceted methods—radiometric dating, stratigraphy, and paleomagnetism—researchers are able to piece together a comprehensive understanding of Earth’s age and the processes that have shaped it throughout its history. Each technique complements the others, contributing to a robust framework for evaluating the timeline of Earth’s development and the origins of life.
The Formation of the Earth: A Cosmic Perspective
The Earth, as we understand it today, originated approximately 4.5 billion years ago during a tumultuous period of cosmic evolution. This era coincided with the formation of the solar system, which began with a vast cloud of gas and dust, known as the solar nebula. Under the force of gravity, this material began to coalesce, leading to the process of accretion. As particles collided and fused together, they formed larger bodies referred to as planetesimals, which continued to aggregate into protoplanets, including our own Earth.
The conditions in the early solar system were marked by extreme heat and chaotic activity. Various celestial phenomena, such as the bombardment of meteorites and the gravitational influence of nearby gas giants, played critical roles in shaping the burgeoning planet. As these protoplanets grew, the force of gravity increased, allowing the Earth to attract even more material. This accumulation of mass resulted in a heated interior, which eventually melted the planet’s surface. This early molten state facilitated the process of planetary differentiation, where denser materials, primarily metals, sank towards the center, forming the core, while lighter materials, like silicates, rose to create the mantle and crust.
The formation of the Earth was not merely a geological process but also a cosmic one, influenced by the dynamics at play in the solar system. As it evolved, the planet’s surface began to cool, leading to the emergence of stable crusts. Volcanic activity during this time contributed to the formation of the atmosphere, setting the stage for the development of life. Understanding the formation of the Earth provides crucial insights into not only our planet’s history but also the broader cosmological processes that govern planetary systems throughout the universe.
Understanding Geological Time Scale
The geological time scale (GTS) serves as a crucial framework in understanding the history of Earth and its life forms. It classifies Earth’s history into divisions known as eons, eras, periods, epochs, and ages, allowing geologists and paleontologists to convey the vast and complex timeline in a more digestible format. The primary divisions start with the Hadean eon, continuing through the Archean, Proterozoic, and Phanerozoic, which represents the era primarily marked by the appearance of abundant life forms.
The Phanerozoic eon is further subdivided into three main eras: Paleozoic, Mesozoic, and Cenozoic. Each of these eras encompasses specific geological events and biological developments. For instance, the Paleozoic era is characterized by the emergence of marine life and the establishment of terrestrial ecosystems, while the Mesozoic era is well-known as the “Age of Reptiles,” where dinosaurs dominated the landscape. The Cenozoic era, the most recent, has seen the rise of mammals and birds, along with significant developments in flowering plants.
The subdivisions of the geological time scale also include various periods, each indicating more specific intervals in Earth’s history. For example, the Jurassic period of the Mesozoic era witnessed the evolution of significant dinosaur species and the flourishing of a variety of plant life. Understanding these divisions is essential for scientists, as they utilize the geological time scale not only to categorize Earth’s history but also to interpret the timing and relationships of events that have shaped the planet over billions of years.
The geological time scale thus provides a structured representation of Earth’s history, enabling researchers to communicate findings regarding both the planet’s physical development and the evolution of life. By analyzing rock strata and fossil records within these time frames, we gain invaluable insights into the chronological changes that occurred throughout Earth’s extensive past.
The Emergence of Life on Earth
The origins of life on Earth remain one of the most fascinating and complex subjects in scientific research. Various hypotheses have been proposed to explain how life began in our planet’s early environment. One prominent theory is the primordial soup theory, which posits that life originated in a warm body of water, where simple organic compounds could form and eventually evolve into more complex molecules. According to this hypothesis, the necessary building blocks for life, such as amino acids and nucleotides, were synthesized from inorganic compounds under conditions prevalent on the early Earth, facilitated by energy sources like ultraviolet light and lightning.
Another theory that has gained traction is the hydrothermal vent theory. This hypothesis argues that life began around hydrothermal vents on the ocean floor, where heated, mineral-rich water provides both the necessary elements and a stable environment conducive to chemical reactions. The temperature and unique chemistry of hydrothermal vents may have allowed for the formation of complex organic structures and the first living organisms, primarily extremophiles that could thrive in such harsh conditions. Research exploring extremophiles has contributed significantly to our understanding of life’s resilience and adaptability.
Beyond these two prominent theories, additional hypotheses also exist, including the RNA world hypothesis and the Panspermia hypothesis. The RNA world hypothesis suggests that self-replicating RNA molecules were precursors to current life, serving as a genetic and catalytic framework. Meanwhile, the Panspermia hypothesis proposes that life may have originated from microorganisms or biochemical compounds that arrived on Earth via comets or meteoroids. Each of these theories contributes to a multifaceted understanding of how life emerged on our planet, highlighting the ongoing exploration of life’s origins and the evolutionary pathways that followed.
Major Events in Earth’s History
The history of Earth is marked by a series of significant events that have shaped its landscape and biological diversity. One of the most pronounced events includes mass extinctions, which have periodically decimated a large fraction of the planet’s biodiversity. The most notable of these, the Cretaceous-Paleogene extinction event approximately 66 million years ago, led to the demise of the dinosaurs and permitted the rise of mammals. This event, alongside others such as the Permian-Triassic extinction, which eradicated nearly 90% of marine species, illustrates the dynamic and often catastrophic nature of life on Earth.
Continental drift is another crucial event in the Earth’s history. Over millions of years, the movement of tectonic plates has dramatically altered the planet’s geography. The process began around 200 million years ago with the break-up of the supercontinent Pangaea. This gradual shifting allowed for the separation and geographic isolation of various species, fostering biodiversity through evolutionary processes. Furthermore, the collision and separation of continents have led to changes in ocean currents and climate, significantly impacting the habitats where life can thrive.
Climate changes throughout Earth’s history have also played a pivotal role in shaping ecosystems. Ice ages, such as the most recent glaciation that began about 2.58 million years ago, have transformed landscapes and influenced species evolution. Conversely, warmer periods led to the expansion of forests and grasslands, allowing for the evolution of different flora and fauna. Each of these climatic shifts corresponds with changes in Earth’s age, providing insight into the planet’s past and foretelling potential future transitions.
Through understanding these major events—mass extinctions, continental drift, and climate changes—we can better appreciate Earth’s complex history and the interplay between geological and biological phenomena. These events not only highlight the age of the Earth but also elucidate the continuous adaptation and survival of life amid ever-changing conditions.
The Relationship Between Earth’s Age and Evolution
The age of the Earth is a fundamental aspect of our understanding of biological evolution and the development of complex life forms. Geological evidence suggests that the Earth is approximately 4.5 billion years old, a timeline that is crucial for the processes of evolution and adaptation to unfold. Evolution, as a scientific theory, posits that species change over time through mechanisms such as natural selection, genetic drift, and mutations. These processes require extensive time scales for significant adaptations to occur.
Key evolutionary milestones, such as the emergence of multicellular organisms, vertebrates, and, eventually, mammals, rely on the long timescales associated with Earth’s history. For instance, the Cambrian explosion, which occurred around 541 million years ago, marked a significant increase in the diversity of life forms, illustrating how evolution can quickly capitalize on the vast periods available in the geological timeline. Further, the rise of land plants approximately 470 million years ago paved the way for terrestrial ecosystems, which in turn influenced the evolution of animals.
The slow but steady changes in environmental conditions, such as climate shifts and geological transformations, have played pivotal roles in shaping evolutionary pathways. For instance, the breakup of supercontinents and the shifting of tectonic plates have both contributed to speciation events by altering habitats and isolating populations. As such, the age of the Earth not only provides a backdrop for these events but also emphasizes the importance of gradual changes in the context of evolutionary biology.
In conclusion, the relationship between Earth’s age and evolution underscores the necessity of long time frames for the development and diversification of life forms. A comprehensive understanding of this relationship illuminates the intricate processes that have shaped the biodiversity that exists today.
Conclusion: Why Understanding the Age of the Earth Matters
Understanding the age of the Earth is pivotal for various realms of science, education, and environmental discourse. The Earth, estimated to be approximately 4.5 billion years old, provides a framework for comprehending geological processes, evolution, and climate changes throughout its long history. By studying the age of the Earth, scientists can gain valuable insights into how natural systems operate, showing the interconnectedness of life, rocks, and atmospheric conditions over immense time scales.
Furthermore, the study of Earth’s age serves as a foundational element in education. It helps foster a comprehensive understanding of natural history within curricula, enabling students to comprehend not only the timeline of life but also the mechanisms behind various scientific phenomena. Instilling this knowledge is crucial, as it empowers future generations to address pressing environmental challenges. Knowledge of the Earth’s age can guide discussions on climate change, resource management, and the sustainability of ecosystems, providing context for the current crises faced globally.
Moreover, recognizing the geological timescale assists scientists and policymakers in predicting future scenarios based on past events. For instance, understanding the patterns of past climate changes allows for better models to forecast how the Earth may react to current human activities. As we grapple with environmental issues such as habitat destruction and global warming, a solid grounding in Earth’s history is essential for developing strategies to mitigate adverse impacts.
In conclusion, the understanding of the Earth’s age is not merely an academic pursuit; it has practical implications that resonate in contemporary discussions on sustainability and climate action. Continuous research and education about the Earth’s deep history remain crucial as we navigate the challenges that lie ahead, ensuring informed decision-making for the well-being of our planet and its future inhabitants.